Li-ion batteries
The need to store energy for portable devices, vehicles, and housing is ever increasing. The transformation from fossil fuels to renewable energy sources needs to hasten to decrease greenhouse gases and limit global warming. The utilization of wind and solar power requires an effective storage system to ensure continuous energy supply as a part of the smart grid. Li-ion battery is considered the best route for many advanced storage applications related to clean electricity due to their high energy density.
Regarding the present Li-ion batteries, one of the limiting factors in their performance is the anode material that most commonly is graphite. Silicon is a promising material for Li-ion battery anodes: By using silicon instead of graphite, the energy density of a battery cell is increased by 30 %. To achieve this, several obstacles must be overcome: First, silicon experiences a volume expansion of 300 % when lithiated. During discharging, the particles tend to fracture and lose contact. Secondly, the volume expansion prevents the formation of a stable electrode-electrolyte interface resulting in a continuous decomposition of the electrolyte. These two reasons cause the limited use of silicon in commercial batteries.
Both problems with silicon material can be avoided by designing optimal porous structures of mesoporous silicon (PSi). The porosity of PSi needs to be high enough to be able to accommodate the volume expansion but also be low enough so that the volumetric capacity/energy density is still higher than that of graphite anodes. Our research is focusing on:
- PSi microfilms to increase Si content in the anode.
- PSi produced from biogenic sources to bring production costs down.
- PSi-carbon nanotube (CNT) hybrids to enhance the charge/discharge rate of the anode.
The image below shows PSi microparticles connected with CNTs to improve the conductivity of the material.
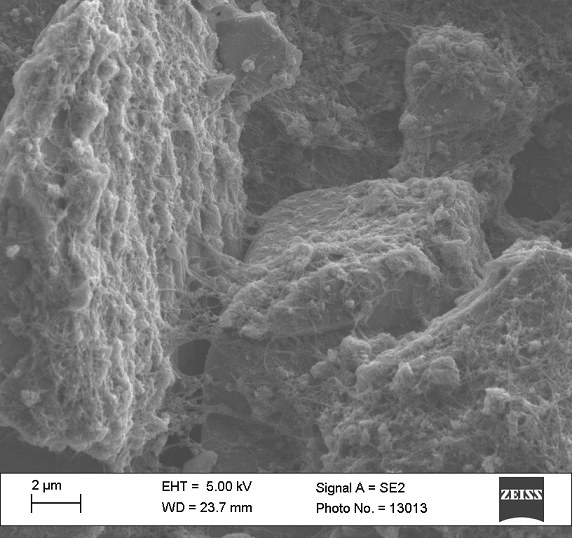