Optical imaging
Optical imaging
Optical imaging uses visible or near-infrared light to investigate the internal properties of biological tissue based on endogenous (e.g. haemoglobin) or exogenous (e.g. dyes) contrast. Particularly, the different absorption spectra of oxygenated and deoxygenated haemoglobin are of the interest. Optical methods offer certain advantages over other clinical imaging methods, for example, they use non-ionising radiation, can provide functional information, and the instrumentation can be built as portable.
We develop computational modelling and image reconstruction methods for optical imaging considering techniques such as diffuse optical tomography and photoacoustic tomography. Furthermore, computational methods for light propagation are studied. The inverse problem is approached in the framework of Bayesian inversion.
Diffuse optical tomography
In diffuse optical tomography (DOT) the optical properties of the medium are estimated based on measurements of near-infrared light made on the surface of the object. DOT belongs to the class of diffuse tomographic methods, which characteristics include e.g. sever ill-posedness of the inverse problem and poor spatial resolution. The provided information, on the other hand, is based on the optical contrast, and thus DOT has potential applications in medical imaging, for example, in detection and classification of breast cancer, functional brain studies, and imaging newborn infant head.
Photoacoustic tomography
In photoacoustic tomography, a nanosecond scale pulse of visible or near-infrared light is used to illuminate the region of tissue of interest. As light propagates within the tissue, it is absorbed by chromophores. The light absorption generates localised increases in pressure resulting in an acoustic wave that propagates through the tissue and can be detected by ultrasound sensors on the surface of the target. In photoacoustic image reconstruction, the initial pressure distribution is reconstructed from the time-varying ultrasound measurements. PAT combines the high spatial resolution of ultrasonic imaging techniques with the benefits of light based measurements. It has successfully been applied to the visualisation of different structures in biological tissue such as human blood vessels, microvasculature of tumours and the cerebral cortex of small animals. The possible applications of the technique include tumour detection, tracking vascularisation, monitoring treatments and small animal imaging. In quantitative photoacoustic tomography (QPAT), one enhances PAT into estimating the concentrations of light absorbing molecules.
Light transport
Radiative transfer is the physical phenomenon of energy transfer in the form of electromagnetic radiation. The propagation of radiation through a medium is affected by absorption, emission, and scattering processes. The radiative transfer equation (RTE) describes these interactions mathematically. RTE has application in a wide variety of subjects including optics, astrophysics, atmospheric science, and remote sensing. Analytic solutions to the radiative transfer equation (RTE) exist for simple cases but for more realistic media numerical methods are required. The approximations of the RTE can be obtained e.g. from non-scattering limit leading to the Beer-Lambert law and for strongly scattering medium leading the diffusion approximation.
The video depicts light pulse propagation in turbid medium with constant optical properties (first row), highly scattering layer (second row) and low-scattering layer (third row). The fluence was solved using a truncated Fourier series approximation of the RTE (first column), a time-dependent RTE (second column), and Monte Carlo simulation (third column). For more information of the numerical approaches, see A. Pulkkinen and T. Tarvainen, “Truncated Fourier-series approximation of the time-domain radiative transfer equation using finite elements”, JOSA A, 30(3):470-478, 2013.
ValoMC – Visible and near infrared light transport in mesh based geometry
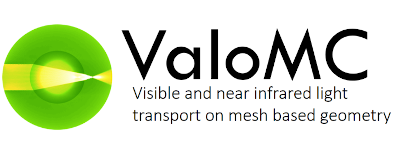
ValoMC is an open source Monte Carlo code that can simulate the passage of visible and near infrared range photons through matter. The simulation geometry is defined using triangular or tetrahedral meshes. The model solves for boundary and volumetric data and supports various boundary conditions, types of light sources, as well as intensity modulated light. While the model is capable of simulating complex measurement geometries, attention is given to ease of use and fast problem set up with a MATLAB (TM) interface. The simulation code is written in C++ and parallelized using OpenMP.
Biomedical Optical Imaging and Ultrasound Laboratory (OPUS)
OPUS laboratory focuses on biomedical optical imaging and ultrasound research. In addition to research of pure optical and ultrasound imaging and therapy methods, the facilities enable hybrid optoacoustic and acousto-optic method development.
Contact
Past and present collaborators
- Professor Simon Arridge, University College London, Department of Computer Science, Centre for Medical Image Computing
- Senior Lecturer Ben Cox, University College London, Department of Medical Physics and Biomedical Engineering
- Professor Adam Gibson, University College London, Department of Medical Physics and Biomedical Engineering
- Professor Arnold D. Kim, University of California, Merced, School of Natural Sciences
- Associate Professor Cosimo D’Andrea, Politecnico di Milano
- Academy Research Fellow Ilkka Nissilä, Aalto University, School of Science